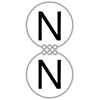
Chloramine Consulting
When bathers enter the pool, there is a time delay between the formation of harmful disinfection byproducts (DBPs), and when the ventilation system can ramp up its exhaust and fresh air intake. In most natatoriums, there is no automated correlation between pool chemistry and ventilation whatsoever, but now it is possible. Let's explore.
Covered in this article:
- How fast are chloramines produced?
- How do HVAC systems adjust for changing conditions?
- Optimize chloramine removal
- Conclusion
How fast are chloramines produced?
Before we can understand how to optimize chloramine removal, we need context. We need to know how they are formed, and how fast they are formed.
When ammonia (or other nitrogen compounds) are oxidized by chlorine, the molecules go through different stages before the oxidation process is completed. For ammonia, the final product is Nitrogen Trichloride (NCl3), more commonly called Trichloramine.
Each successive stage takes an increasing amount of time. In practical terms, it all happens pretty quickly, though the off-gassing of trichloramine can take longer than its production. With agitated water–like swim practice, splash features or other turbulence–the rate of off-gassing is faster.1
Related: Pool Chemistry pt. 2: Combined Chlorine
The rate of chloramine production is important because it requires us to adapt our strategy in managing these harmful byproducts. If chloramines took hours or days to produce, circulation rates would be fast enough for secondary systems like UV and Ozone to prevent air quality problems. But that's not the case. These byproducts can release into the air before the secondary system has a chance to see it, since secondary systems are point-of-contact, and are at the mercy of the circulation rate–also called the turnover rate.
A typical commercial pool turnover rate is between 4-6 hours. Chloramines can off-gas in minutes. Therefore, chloramine vapor is an air problem for the HVAC system to manage.
What factors impact the rate of chloramine production?
If you can slow down the production rate of chloramines without sacrificing too much on disinfection, that can be an advantage. The best way to thwart chloramine production, of course, is to prevent nitrogen from getting in your water in the first place. But since bathers introduce ammonia and urea to the water, it's a safe assumption that you have ammonia (or other nitrogen compounds) in your water.
That said, a few factors can influence the speed of the chlorine reactions that create chloramines. In particular, pH, water temperature, and the concentration of hypochlorous acid (%HOCl).
Related: Pool chemistry, part 1: Chlorination
pH
Water temperature
%HOCl
Let's expand upon the percentage of hypochlorous acid. Indeed, pH controls the "strength" of chlorine (%HOCl) if no CYA is present in the water. That's because when chlorine dissociates, it becomes an equilibrium between HOCl and OCl-.
HOCl ⇌ H+ + OCl-
Hypochlorous Acid dissociates into Hydrogen and Hypochlorite Ion
The higher the pH, the higher the percentage of OCl-, which is over 100x slower and weaker than HOCl as a disinfectant and oxidizer. Keep that in mind, because what we're about to tell you comes with an important caveat...
Aside from the obvious main factor–the amount of nitrogen compounds in your water–the percentage of HOCl is the driving factor of chloramine production. Reducing the HOCl percentage will slow the production rate of chloramines, which can buy you time for a secondary system to help more. And there are a few ways to reduce the %HOCl. You can raise the pH, or you can add a small amount of CYA.
The caveat here is we need HOCl as our primary disinfectant in the pool! So we cannot drop our %HOCl without consequences. There is a fine line between benefit and detriment. And we are not advocating for CYA on an indoor pool, we are just mentioning it because it is an option. The better option is to keep your free chlorine maintained as low as possible (usually between 1-2 ppm), and your pH between 7.5-7.8.
Wait, what?!
Did you see that? 7.5-7.8 pH? Yes, we know that's higher than most operators aim for...but hear us out.
-
-
- Higher pH means a lower %HOCl, which slows chloramine production. This gives secondary systems more time to help supplement chlorine against forming chloramines.2
- Higher pH means a slower rise in pH, because the pH naturally rises with the loss of carbon dioxide. The higher your pH, the closer the water is to its 'pH ceiling' (pH(eq)). To learn more about this concept, read this.
- Since most health departments require 7.8 or lower pH, setting your pH to 7.5 or even 7.6 (instead of 7.2-7.4) means a smaller pH rise (not just slower), less acid consumption, and less sodium bicarb too. Wins all around.
-
pH and chlorine strength (%HOCl)
If someone is telling you that the water needs to be below 7.6 pH to have effective sanitizer, consider the objective here. Sure, lower pH will yield a higher %HOCl, but outdoor pools operate with cyanuric acid (CYA) all the time, and their sanitization can be totally fine, assuming the water is not overstabilized.
Here's a chart that shows the strength of chlorine (expressed as %HOCl) on the same pool, with and without 30 ppm of CYA:
Notice the red line on the left chart, with no CYA. The pH clearly dictates the %HOCl, and lower pH means stronger chlorine. But on the right chart, with 30 ppm CYA, where is the red line now?
As you can see, the %HOCl is below 3%, and shows little-to-no difference between 7.0 and 8.5 pH. That's because the majority of chlorine is bound to CYA, and that molecule–called an isocyanurate–is not pH dependent like HOCl is. So you still have plenty of chlorine, it's just temporarily bound to CYA.
The point is–getting back to our pH conversation–you don't necessarily need a super high percentage of HOCl to have effective disinfection. Sure, you need it, but if you can slow the production of chloramines while still having effective disinfection in the water, that allows more time for a secondary system like UV, Ozone or AOP to destroy more chloramines.
It's just something to consider. Again, we're not advocating for CYA in indoor pools, but Richard Falk's research on the topic has merit, and is worth reading.3
So now that we have covered the rate of chloramine production in the water, let's shift gears and talk about the air side of the equation. What's going on above the water in the natatorium?
How do HVAC systems adjust for changing conditions?
There's a huge difference between a busy swim practice and when the pool is closed at night. But the pool dehumidification system never sleeps. So how does it adjust for the differences in demand?
Related: Swimming Pool Air Quality Resources
Timing automation (modes)
Conventional systems are usually operated by a programmed timer that tells the system when to ramp up and ramp down. And by ramp up and down, we mean open up the dampers for more outside air (OA), and proportionally increase exhaust air (EA) so that more dilution flows through the natatorium when the pool is busy. There are several modes, and each manufacturer may have different names for them. Generally speaking, there are occupied, unoccupied, and purge modes.
Manual settings
These modes can also be manually toggled on most systems. Say the air quality is lousy and lifeguards are coughing, and the facility needs to be aired out. The building manager can turn the HVAC system to purge mode and introduce a LOT more outside air to dilute the space. The problem with this, especially in winter, is the cost of heating outside air can be expensive. Think of the energy it takes to turn 20ºF air into 84ªF air. It's not cheap.
VOC meters
Another mechanism for signaling the pool dehumidifcation unit (PDU) to adjust its OA/EA is with something called a VOC meter. A VOC meter measures Volatile Organic Compounds. This is largely experimental at this phase, but has shown to recognize when air quality declines. It can send a signal to the controller that adjusts the PDU accordingly.
The main problem with VOC meters in indoor pools is reliability. They work well in most environments, but the metal components inside the device are prone to rapid corrosion from airborne chloramines (go figure). So they don't last long, in our experience. It's a cool idea though, while it lasts. VOC meters were the first iteration of 'on-demand chloramine control'. In other words, they send a demand-based signal to the PDU, instead of a timer or manual override by a human operator. But now, there's a much more reliable, real-time signal that is not subject to corrosion from chloramines....
Combined chlorine/ORP controller signal
We will give credit where credit is due. The first person we know of who came up with this clever system is Tom Carrico of Carrico Aquatic Resources in Wisconsin. Its brilliance is in its simplicity. Have the pool chemical controller send a signal to the PDU's controller, or the exhaust fan's variable frequency drive (VFD). The pool chemical controller needs to be able to read real-time combined chlorine and ORP.
When the combined chlorine level rises, and/or the ORP drops, that indicates there's a bather load right now. The controller sends a signal to the HVAC system (or its exhaust fan) to ramp up. In the case that the PDU is older and does not have such capability, you can install a VFD for the exhaust fan, send the signal to that, and use a pressure sensor in the PDU to adjust the OA damper accordingly. We're not engineers, but we have seen this done in several different ways, and a good mechanical contractor can figure this out. It's beautifully simple.
How to optimize chloramine removal
There are three basic principals for addressing an indoor pool air quality problem:
-
-
- Identify and address (or prevent!) any and all sources of nitrogen getting into the water. Look for deck cleaners especially, and encourage patron hygiene programs like showering before swimming, and not peeing in the pool. And good luck with those last two.
- Supplement chlorine against the oxidant demand. Specifically address nitrogen compounds and non-living organics. A reliable secondary system is a must for indoor commercial pools, but will be point-of-contact without residual. For a residual supplement that circulates along with chlorine in the water, consider NSF-50 Certified enzymes to remove non-living organics and oils. Both these strategies dramatically reduce chlorine demand.
- Have a source-capture exhaust system integrated with a properly-sized pool dehumidifier. Source-capture exhaust pulls air from the bottom of the room, where the heavier-than-oxygen chloramines are concentrated. When designed correctly–and plenty of engineers have tried (and failed) to design their own–these exhaust systems are effective. They need good supply airflow and an integration with the PDU for air balance, but once they're installed, the only maintenance on them is a fan.
-
If you can nail all three, your air quality will be exceptionally good. Even if you struggle with these, you can improve indoor air quality by focusing in those three areas.
Most pools cannot nail all three, however. Especially with existing facilities, it can be a serious challenge to modify the room and get creative on how to get chloramines out. But that's where we come in, with our facility evaluation. Having evaluated over 300 natatoriums, we know what we're looking for, and advise the mechanical engineer and contractor(s) of your choice on how to solve the problem.
Conclusion
Address the three points listed above, coordinate systems with on-demand chloramine detection if you can, and do what you can to slow the production of chloramines in the first place. If you do these things, your air quality will improve. It's not if, it's by how much?
1 This study, published by NIH, found that the mass transfer of trichloramine from liquid to gas takes about 20 hours to 5.8 days. They wrote: "Also the mass transfer of NCl(3) from water to the gas phase which was calculated by the Deacon's boundary layer model could be shown as a relatively slow process. Mass transfer would take 20 h or 5.8 d for a rough or a quiescent surface of the water, respectively. This is much more than a typical turnover rate of 6-8 h of a treatment cycle of a 25 m swimming pool. Therefore processes to remove NCl(3) and its precursors can help to minimize the exposure of bathers." But this is a study from a lab, and it conflicts with our experience in the field with hundreds of real natatoriums. If their findings were true across the board, indoor air quality problems should not exist if a pool has a working UV or Ozone system. Yet, IAQ problems persist. In our opinion, empirical evidence outweighs this lab test significantly, because the "Deacon's boundary layer model" is only theoretical. Our experience is real. Just ask anyone who has been in an indoor pool for a few hours with a swim practice or meet going on.
2 We say 'forming chloramines' here because UV systems are not oxidizers, but they can destroy combined chlorine. That is, chlorides bound to nitrogen compounds. Ozone and AOP, on the other hand, can destroy chloramines and the nitrogen compound precursors because they are powerful oxidizers.
3 The Chlorine/Cyanuric Acid relationship, and implications for Nitrogen Trichloride. By Richard A. Falk..
Submit a Comment